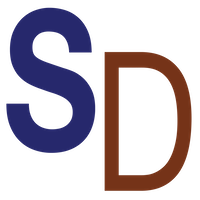
In what they labeled a “surprising” finding, Johns Hopkins Medicine researchers studying bacteria from freshwater lakes and soil say they have determined a protein’s essential role in maintaining the germ’s shape. Because the integrity of a bacterial cell’s “envelope” or enclosure is key to its survival, the finding could advance the search for new and better antibiotics.
The research, described August 15 in the journal mBio, suggests that loss of a protein called OpgH in a widely studied bacterium known as Caulobacter crescentus creates a cascade of activity that disrupts the bubble-like cell envelope protecting the bacterium, resulting in the cell’s death. OpgH is an enzyme that creates glucose-containing molecules known as osmoregulated periplasmic glucans, or OPGs, which fill up the gelatinous in-between spaces of the protective cell envelope.
“In our experiments, when we get rid of the protein OpgH in Caulobacter bacteria, which halts production of OPG sugar molecules, the bacteria can’t survive,” says senior study author Erin Goley, Ph.D., professor of biochemistry at the Johns Hopkins University School of Medicine.
While Caulobacter crescentus bacteria themselves are not generally thought to cause diseases, OPGs found abundantly in gram-negative bacteria — bacteria in an enclosed shell-like membrane — play a role in antibiotic resistance and disease outcomes.
As a result, efforts to better understand the role of the sugar molecules in gram-negative bacteria, including Caulobacter, are seen as a way to aid in the development of new drugs that target disease-causing bacteria that have OPGs, including Brucella, Pseudomonas, Salmonella and E.coli.
If it’s true that the proteins that make or modify these sugar molecules are essential to bacterial survival, Goley notes, they could be good drug targets for antibiotics themselves. Or, in organisms where OPGs are not essential, a drug that targets some part of the OPG pathway might sensitize the cells to existing antibiotics, she says.
In this study, scientists used a molecular tool called an inducible promoter, dialing down the presence of the OpgH protein in Caulobacter to observe the effects on the shape of the bacteria cell and how loss of the OpgH protein affects a signaling pathway known as CenKR, which identifies and repairs problems in the cell envelope. They also manipulated the cell to make too much of the protein CenR, thus hyperactivating the CenKR pathway responsible for regulating the shape of the cell envelope.
After dialing up or down the OpgH or CenR proteins, scientists placed the bacteria cells on a pad of gel that prevented them from moving. Then, researchers used a specialized microscope to observe their shapes and activities.
“We found that they became misshapen as we dialed down the OpgH protein and halted production of sugar OPG molecules, or hyperactivated the CenKR signaling pathway that maintains the cell envelope,” Goley says.
“Then we also looked at where some of the molecular players that helped to grow the cell and keep the shape of the cell were located,” she says. “The molecular players were not in the correct locations, suggesting that OpgH and CenR are integral to maintaining the cell’s shape.”
Once the cell envelope loses its shape, all of the bacteria eventually burst open and die, Goley says.
“We established a model for how either depleting OPGs or activating the signaling pathway affects cell shape and growth,” Goley says.
While characterizing the sugar molecule’s role in Caulobacter’s cell structure is an important first step, Goley cautioned that “it will take some time to develop a complete picture about how they function across gram-negative species of bacteria.”
In Caulobacter, the sugar molecules resemble closed rings, and in E. Coli, they look like trees, with branches sticking off of chained structures. Understanding their shape and all of the decorations that associate with the molecules can help researchers characterize the cell envelope, she says.
“In the next phase of research, we hope to investigate all of the enzymes that make, decorate and break down these molecules — so we can get a full picture of their metabolism and how they maintain the cell envelope,” Goley says. “Once we uncover how these enzymes function, that’s great, because those are things drugs can target.”
Other scientists who contributed to this research include co-first authors Allison Daitch and Erika Smith, both recent doctoral graduates in Goley’s lab at Johns Hopkins Medicine, and now at the Biomedical Advanced Research and Development Authority at the U.S. Department of Health and Human Services and the National Institutes of Health, respectively.
Funding for the research was provided by the National Institute of General Medical Science (R35GM136221, T32GM007445).