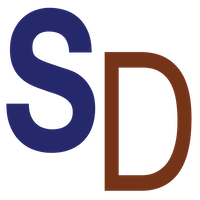
One in every three FDA-approved drugs targets a single superfamily of receptors dotting the surfaces of human cells. From beta blockers to antihistamines, these essential, life-saving medications trigger winding biochemical pathways, via these receptors, to ultimately prevent a heart attack, or stop an allergic reaction in its tracks.
But scientists have learned that their story is much more complicated than initially believed — a number of these drugs are in fact targeting a complex composed of one receptor and one associated protein. Now, a new study in Science Advances introduces a novel approach to mapping the interactions between 215 such receptors and the three proteins that they form complexes with. The findings dramatically expand understanding of these interactions and their therapeutic potential.
“On the technical side, we can now study these receptors at unprecedented scale,” says first author Ilana Kotliar, a former graduate student in Rockefeller’s Laboratory of Chemical Biology and Signal Transduction, headed by Thomas P. Sakmar. “And on the biological side, we now know that the phenomenon of these protein-receptor interactions is much more widespread than originally thought, opening the door to future investigations.”
Uncharted territory
This family of receptors are known as GPCRs, or G protein-coupled receptors. Their accessory proteins are known as RAMPs, short for receptor activity-modifying proteins. RAMPs help transport GPCRs to the cell surface and can vastly alter how these receptors transmit signals by changing the receptor’s shape or influencing its location. Because GPCRs seldom exist in a vacuum, identifying a GPCR without accounting for how RAMPs might influence it is a bit like knowing the menu of a restaurant without checking its hours, address or delivery options.
“You could have two cells in the body in which the same drug is targeting the same receptor — but the drug only works in one cell,” says Sakmar, the Richard M. and Isabel P. Furlaud Professor. “The difference is that one of the cells has a RAMP that brings its GPCR to the surface, where that the drug can interact with it. That’s why RAMPs are so important.”
Knowing this, Sakmar and colleagues were determined to develop a technique that would allow researchers to parse out each RAMP’s effect on every GPCR. Such a comprehensive map of GPCR-RAMP interactions would supercharge drug development, with the added benefit of possibly explaining why some promising GPCR drugs mysteriously haven’t panned out.
They hoped that such a map would also contribute to basic biology by revealing which natural ligands several so-called “orphan” GPCRs interact with. “We still don’t know what activates many GPCRs in the human body,” Kotliar says. “Screenings may have missed those matches in the past because they weren’t looking for a GPCR-RAMP complex.”
But wading through every GPCR-RAMP interaction was a daunting task. With three known RAMPs and almost 800 GPCRs, searching through every possible combination was impractical, if not impossible. In 2017 Emily Lorenzen, then a graduate student in Sakmar’s lab, began a collaboration with scientists at the Science for Life Laboratory in Sweden and Sweden’s Human Protein Atlas Project to create an assay capable of screening for GPCR-RAMP interactions.
Hundreds of experiments at once
The team started by coupling antibodies from the Human Protein Atlas to magnetic beads, each pre-colored with one of 500 different dyes. These beads were then incubated with a liquid mixture of engineered cells expressing various combinations of RAMPs and GPCRs. This setup allowed researchers to simultaneously screen hundreds of potential GPCR-RAMP interactions in a single experiment. As each bead passed through a detection instrument, color coding was used to identify which GPCRs were bound to which RAMPs, enabling high throughput tracking of 215 GPCRs and their interactions with the three known RAMPs.
“A lot of this technology already existed. Our contribution was an enabling technology built upon it,” Sakmar says. “We developed a technique to test for hundreds of different complexes at once, which generates a huge amount of data, and answers many questions simultaneously.”
“Most people don’t think in multiplex terms. But that’s what we did — 500 experiments at once.”
While this work is the culmination of a team effort over a long period of time, Kotliar made herculean efforts to drag it across the finish line — shuttling samples and scarce reagents back and forth from Sweden in rare travel windows during COVID.
It paid off. The results provide a handful of long-awaited resources for GPCR researchers and drug developers: publicly available online libraries of anti-GPCR antibodies, engineered GPCR genes and, of course, the mapped interactions. “You can now type in your favorite receptor, find out what antibodies bind to it, whether those antibodies are commercially available, and whether that receptor binds to a RAMP,” Sakmar says.
The findings increase the number of experimentally identified GPCR-RAMP interactions by an order of magnitude and lay the groundwork for techniques that could help detect combinations of GPCRs and identify harmful autoantibodies. “Ultimately, it’s a technology-oriented project,” Sakmar says. “That’s what our lab does. We work on technologies to advance drug discovery.”