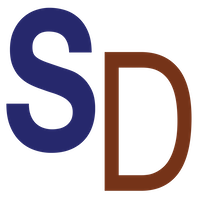
Our ability to see starts with the light-sensitive photoreceptor cells in our eyes. A specific region of the retina, termed fovea, is responsible for sharp vision. Here, the color-sensitive cone photoreceptors allow us to detect even the smallest details. The density of these cells varies from person to person. Additionally, when we fixate on an object, our eyes make subtle, continuous movements, which also differ between individuals. Researchers from the University Hospital Bonn (UKB) and the University of Bonn have now investigated how sharp vision is linked to these tiny eye movements and the mosaic of cones. Using high-resolution imaging and micro-psychophysics, they demonstrated that eye movements are finely tuned to provide optimal sampling by the cones. The results of the study have now been published in the journal eLife.
Humans can fixate their gaze on an object to see it clearly thanks to a small region in the center of the retina. This area, known as the fovea (latin for “pit”), is made up of a tightly packed mosaic of light-sensitive cone photoreceptor cells. Their density reaches peaks of more than 200,000 cones per square millimeter — in an area about 200 times smaller than a quarter-dollar coin. The tiny foveal cones sample the portion of visual space visible to the eye and send their signals to the brain. This is analogous to the pixels of a camera sensor, with millions of photo-sensitive cells spread across their surface.
However, there is an important difference: unlike the pixels of a camera sensor, the cones in the fovea are not uniformly distributed. Each eye has a unique density pattern in their fovea. Additionally, “unlike a camera, our eyes are constantly and unconsciously in motion,” explains Dr. Wolf Harmening, head of the AOVision Laboratory at the Department of Ophthalmology at UKB and a member of the Transdisciplinary Research Area (TRA) “Life & Health” at the University of Bonn. This happens even when we are looking steadily at a stationary object. These fixational eye movements convey fine spatial details by introducing ever-changing photoreceptor signals, which must be decoded by the brain. It is well known that one of the components of fixational eye movements, termed drift, can differ between individuals, and that larger eye movements can impair vision. How drift relates to the photoreceptors in the fovea, however, and our ability to resolve fine detail has not been investigated until now.
Using High-Resolution Imaging and Micro-Psychophysics
This is precisely what Harmening’s research team has now investigated by using an adaptive optics scanning light ophthalmoscope (AOSLO), the only one of its kind in Germany. Given the exceptional precision offered by this instrument, the researchers could examine the direct relationship between cone density in the fovea and the smallest details we can resolve. At the same time, they recorded the tiny movements of the eyes. To do this, they measured visual acuity of 16 healthy participants while performing a visually demanding task. The team tracked the path of the visual stimulus on the retina to later determine which photoreceptor cells contributed to vision in each participant. The researchers — including first author Jenny Witten from the Department of Ophthalmology at UKB, who is also a PhD student at the University of Bonn — used AOSLO video recordings to analyze how the participants’ eyes moved during a letter discrimination task.
Eye Movements are finely tuned to Cone Density
The study revealed that humans are able to perceive finer details than the cone density in the fovea would suggest. “From this, we conclude that the spatial arrangement of foveal cones only partially predicts resolution acuity,” reports Harmening. In addition, the researchers found that tiny eye movements influence sharp vision: during fixation, drift eye movements are precisely aligned to systematically move the retina synchronized with the structure of the fovea. “The drift movements repeatedly brought visual stimuli into the region where cone density was highest,” explains Witten. Overall, the results showed that within just a few hundred milliseconds, drift behavior adjusted to retinal areas with higher cone density, improving sharp vision. The length and direction of these drift movements played a key role.
According to Harmening and his team, these findings provide new insights into the fundamental relationship between eye physiology and vision: “Understanding how the eye moves optimally to achieve sharp vision can help us to better understand ophthalmological and neuropsychological disorders, and to improve technological solutions designed to mimic or restore human vision, such as retinal implants.”
Funding: This work was supported by the Emmy Noether Program of the German Research Foundation (DFG); the Carl Zeiss Foundation (HC-AOSLO); Novartis Pharma GmbH (EYENovative research award), and the Open Access Publication Fund of the University of Bonn.